Dilpreet Kaur, a PhD candidate at ICRAR-Curtin, has recently used telescopes in Australia and India to conduct research relating to detecting gravitational waves using an array of pulsars.
Ever since their discovery in 1967, pulsars have amazed astronomers with their extreme physical conditions and environments as well as their wide-ranging applications for physics.
Fast-spinning, highly magnetised, tiny but very dense stars, pulsar signals appear in the form of trains of regular pulses, when their radio emission beams point toward earth; they are arguably amongst the most widely exploited astrophysical objects.
Pulsars have been used to test and further advance a number of physical theories since their discovery; a high-profile application of these fascinating objects is the search for the signatures of ultra-low-frequency gravitational waves, and is a key science driver for the Square Kilometre Array (SKA) project that is now closer to construction.
Ground-based Gravitational-wave detectors are restricted in the range and types of objects they can detect given the size of such detectors.
For instance, the 4 km arms of the Laser Interferometer Gravitational-wave Observatory (LIGO) located in the United States is most sensitive to gravitational waves in the kiloHertz to Hertz range of frequencies, and these are produced by mergers of pairs of black hole roughly 30 to 100 times more massive than our Sun.
But to detect even more massive mergers and events, like the mergers of large galaxies as they formed and evolved in the early stages of the universe, we need to go into the nanohertz range where the wavelength is much longer, and hence requires more sensitive, and large, detectors than we currently have.
The good news is we don’t have to build a colossal detector through the planet or in orbit.
The “building blocks” for our detector already exist in nature, and are waiting to be exploited: they are millisecond pulsars, i.e. pulsars that spin at rates of several hundred times per second, the fastest one spinning at a rate faster than that of a kitchen blender!
Pulsars are like interstellar lighthouses for astronomers, with millisecond pulsars spinning at rates of several rotations a second, and therefore can be precisely timed, often with precisions of a millionth of a second, or even down to ten-millionth, in the best-case scenario.
These repeating, and astonishingly regular, flashes of radio light can be used to build a Pulsar Timing Array (PTA) – a celestial distribution of pulsars that can be regularly timed with very high precisions, in our quest to detect ultra-low-frequency gravitational waves.
With such highly predictable intervals of these pulses, it is possible correlate timing data sets from different pulsars (and from different telescopes) to search for the signatures of gravitational background – something produced by millions of supermassive black-hole pair mergers, as the galaxies harbouring them merged in the early history of the universe.
By monitoring several of these pulsars over many years, astronomers are hoping to detect the telltale signature of this gravitational wave background pervading through our universe. The signal strength is however expected to be much fainter due to the very large distances, of the order of billions of light years.
But detecting these ultra-low frequency waves is easier said than done.
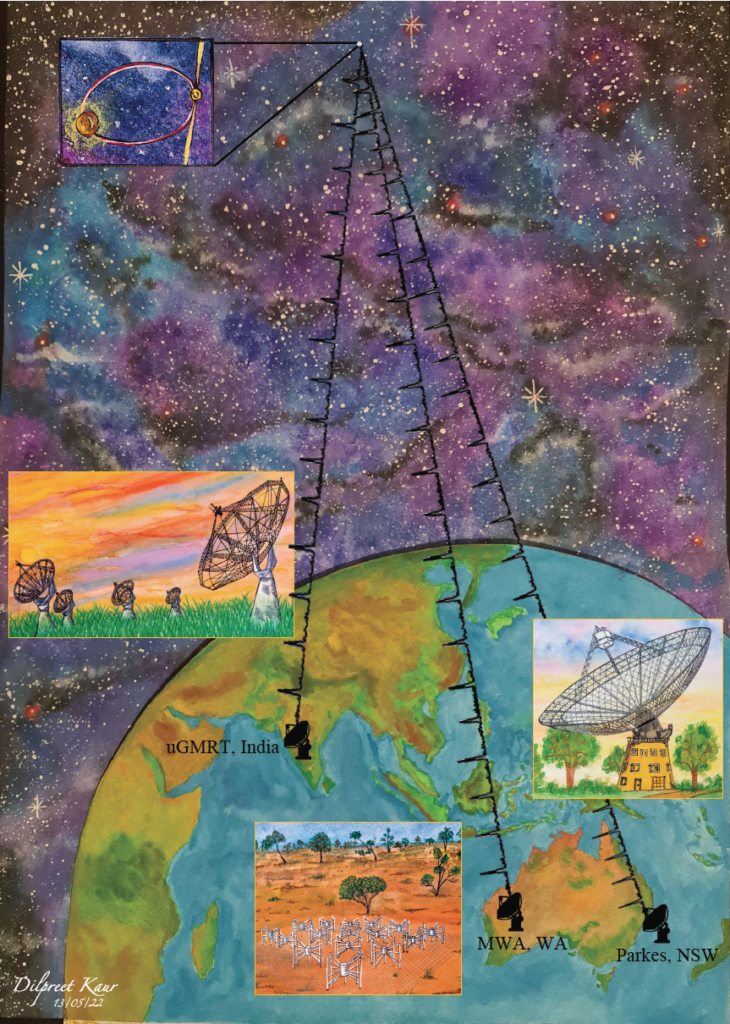
From Earth, pulsar signals have their arrival times altered depending on the nature of the interstellar medium (ISM) between us and them, making monitoring these celestial objects quite tedious.
The ISM is weird and complex; it behaves subtly differently from pulsar to pulsar, and can even give rise to effects such as chromatic (i.e. frequency-dependent) dispersion in the arrival times of pulsar signals as they interact with the free electrons on their journey to the telescope.
This dispersion effect manifests as a parabolic sweep in arrival times at the telescope, and even though the density of electrons is incredibly small, the 1000s of light years the pulsar signals travel through can amount to measurable changes in the signal’s arrival times.
In principle, this effect could be precisely measured with advances in telescope instrumentation, but it’s only one of several subtleties that need to be accounted for.
Due to pulsars whizzing in space with high velocities of the order of a million km per hour, and the arrival times being measured with a precision better than a microsecond, observations separated in time will probe different parts of the ISM; in principle, they may also change depending on where they are located at the time – in relation to earth’s rotation and our orbit, resulting in slightly varying readings of the ISM called Dispersion Measure or DM, which is the total electron content in the path between the pulsar and telescope.
Based on early observations of pulsars with the Murchison Widefield Array (MWA), a team led by ICRAR-Curtin PhD candidate Dilpreet Kaur undertook concerted multi-telescope observing campaigns of one of the high-priority pulsars targeted for Pulsar Timing Arrays.
This pulsar, catchily named PSR J2241–5236, spins at a rate of more than 450 times per second, and on each rotation, its signal is visible to us for about 5% of its rotation period – roughly ~150 microseconds.
Using data collected at the MWA in Western Australia, the Giant Metre-wave Radio Telescope in India, and the Parkes (Murriyang) radio telescope in New South Wales, within a span of less than 24-hours, Dilpreet & team were able to measure, calibrate, and analyse these arrival time perturbations and obtain high-precision measurements of DM, to reveal a clear frequency dependence.
These results present the first definitive proof predictions that the Dispersion Measure can vary with the frequency the observer uses to detect these stellar objects.
It’s part of a long process of understanding how pulsar signals vary in their arrival times, and learn how to mitigate these variations as we march toward the ultimate goal of PTAs, i.e. the detection of nanohertz-frequency gravitational waves.
The analysis and results reporting this was published in the Astrophysical Journal Letters last month.